Spectral imaging, also known as imaging spectroscopy, refers to methods and devices for acquiring a complete light spectrum for each point in the image of a scene. Among them, hyperspectral imaging acquires the full continuous spectrum of light for each point (x,y) of the scene, resulting in a three-dimensional data-cube as a function of (x,y,w), where w is the frequency.Starting from the cube it is possible either to extract the full spectrum from a pixel of the image, or visualize the image at a specific frequency. From the full spectral information, numerical methods enable extracting quantitative parameters related to chemical and physical properties of the imaged objects. Hyperspectral imaging hence provides much richer information with respect to standard imaging, enabling to identify materials or detect dynamical processes.
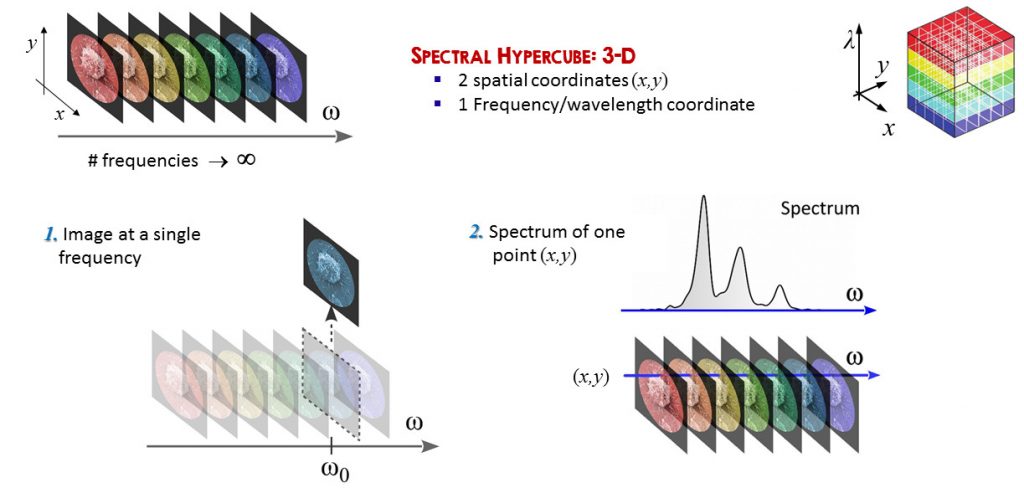
Spectral imaging has been applied to a wide range of scientific investigations and at various scales, such as remote sensing, pigment determination in biology, medicine, coastal ocean imaging, water analysis, agriculture, cultural heritage and archaeology, just to cite a few.
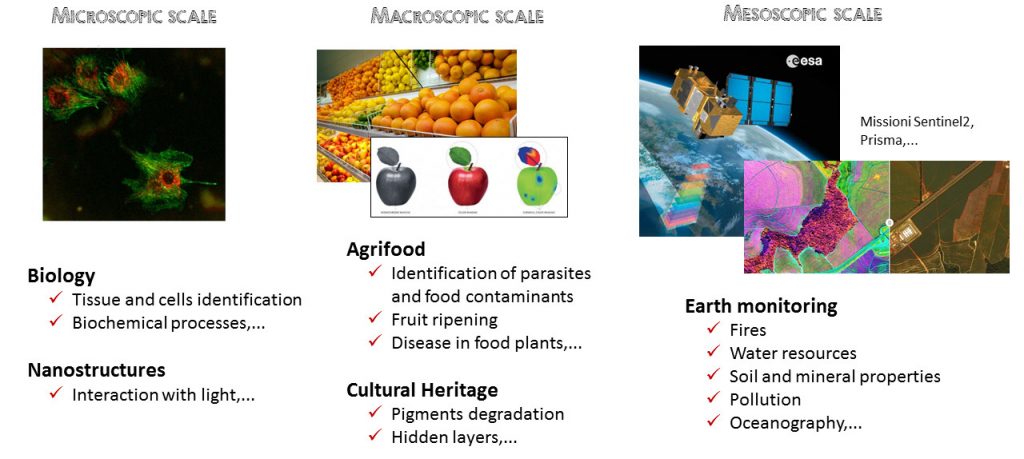
Hyperspectral imaging can be obtained by a variety of techniques, which are used also in commercial devices. However, these techniques have some flaws, such as the low throughput; this requires to illuminate the object with strong light, which could alter the measurement.We have realized and patented a novel Hyperspectral imaging system, which is based on an innovative technology, initially developed in our labs for femtosecond 2D spectroscopy [1]. The hyperspectral system is based on the Fourier-transform approach [2], a powerful technique which is characterized by large throughput, broad flexibility in terms of spectral coverage and resolution and extreme robustness [3, 4].
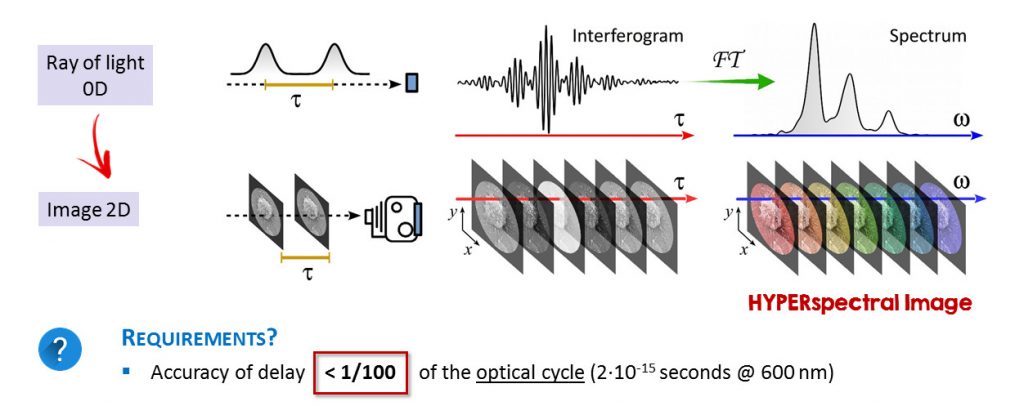
Our compact hyperspectral system is able to acquire spectral reflectance and fluorescence images with high sensitivity, broad spectral coverage and high spectral resolution.
Experimental works are focused on:
Cultural Heritage
Conservation science takes care of protecting artworks, architecture and cultural works in general through the use of scientific methods. The richness of colors and the complexity of artworks call for a method capable of investigating, with a single instrument, heterogeneous materials like stones, pigments, textiles and many others. This makes hyperspectral imaging an ideal tool for conservation, since it can effectively reveal stability issues in paintings, sculptures and artworks in general. This is mainly true for color fading, darkening of the surface of artworks due to environment pollution or other subtle alterations ascribable to inappropriate conservation conditions. With our camera we acquired hyperspectral images of samples ranging from Egyptian artworks [3] to paintings attributed to Michelangelo Buonarroti [6], to artistic glass windows for the characterization of their nanoparticles composition [3].
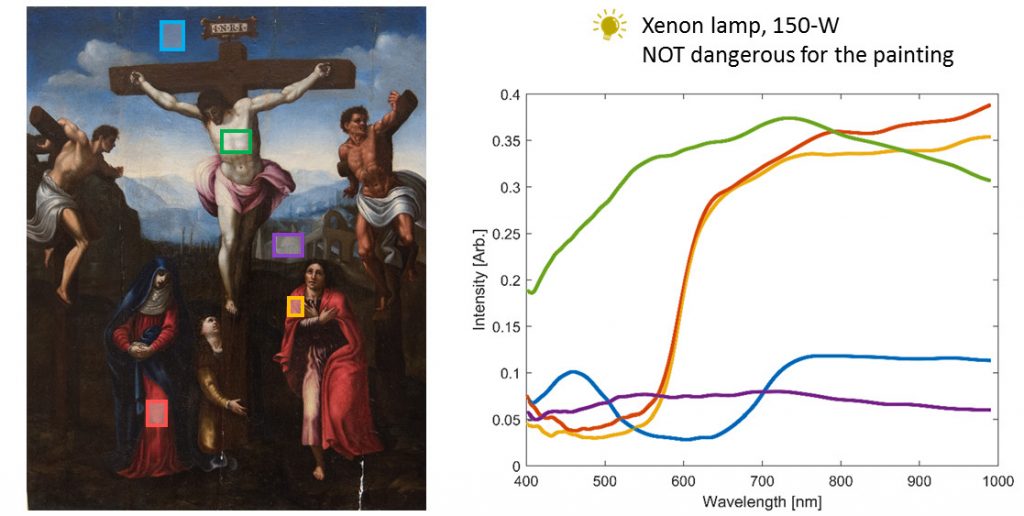
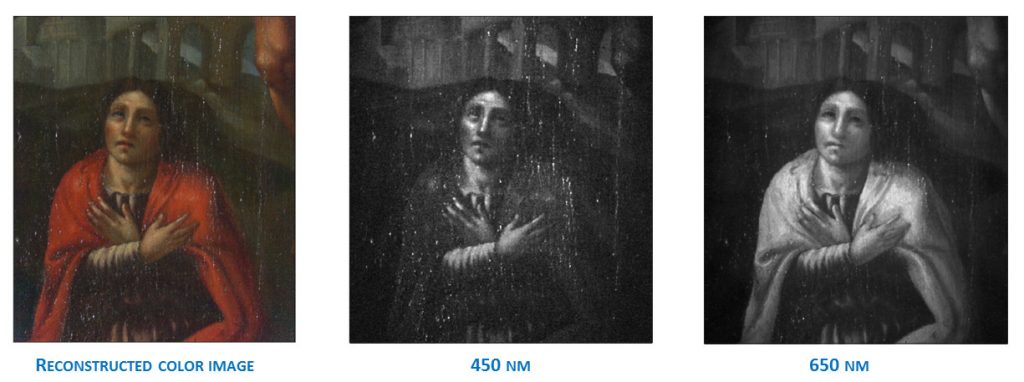
The measurement of a stained glass window, whose plates typically exhibit strong absorption bands located at well-defined wavelengths, was performed at Santo Spirito Church in Milan (Italy), designed in 1969 by A. M. Nardi (1897-1973). The window was back-illuminated by natural sunlight, and the camera recorded the transmitted light. From the data-cube, we extracted a subset of images at selected wavelengths; as expected, each tile absorbs at specific spectral bands. Automatic image k-means segmentation of the blue tiles allowed their separation into 5 classes with distinct spectral transmission and hence material composition, despite their similar visual appearance. In particular, we identified two blue tiles with negligible infrared transmission, which probably are a late replacement.
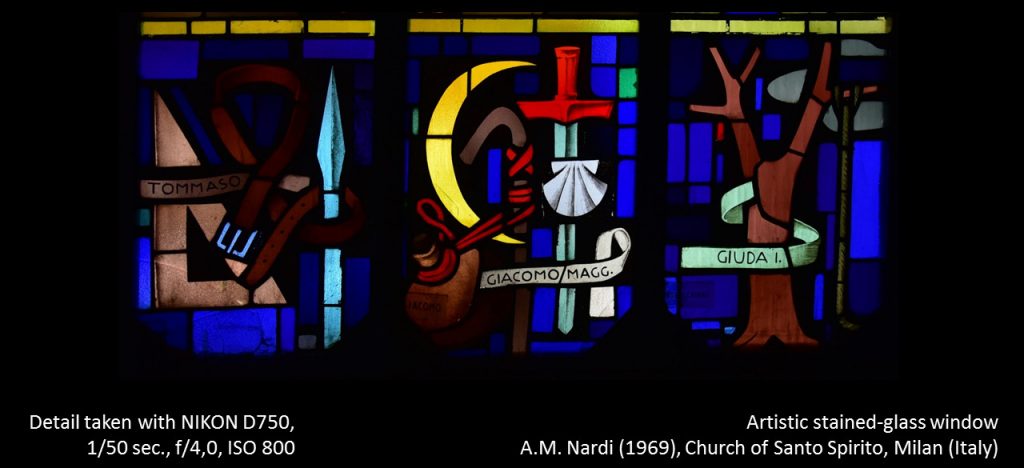
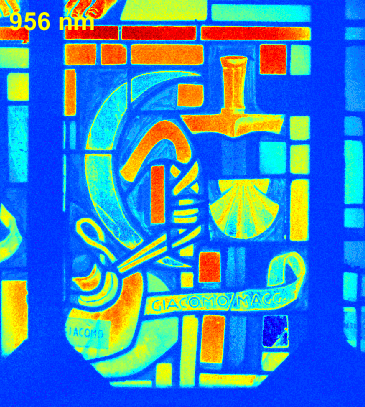
Microscopy of cells and nanostructures
Our Hyperspectral setup could also be applied to microscopy, to perform fluorescence imaging of cells and nanostructures. We tested our setup by imaging various samples: an RGB AMOLED display, doubly labeled cells, and nanostructures.
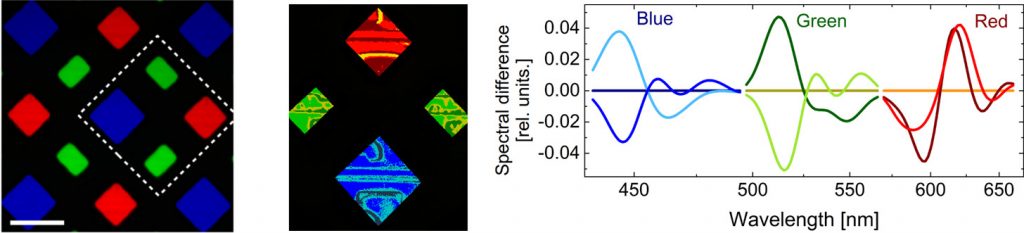
The microscope enables also detecting the constituents of cells; this is obtained by staining the cell, and measuring the fluorescence emission spectrum after UV illumination.
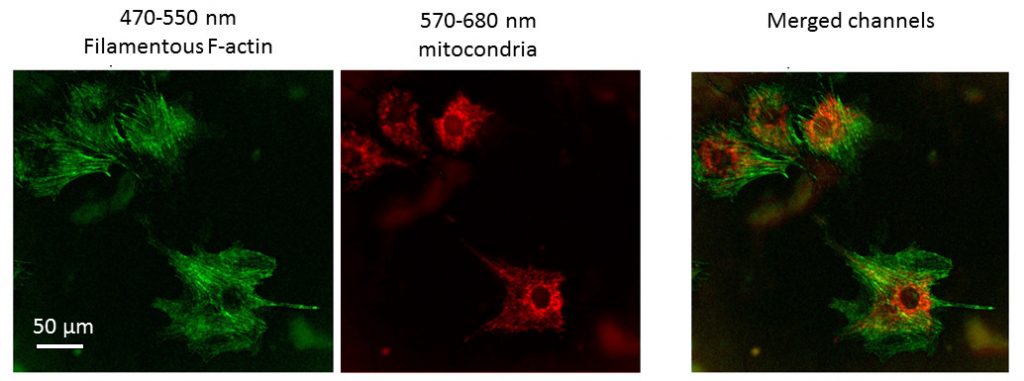
References
https://www.facebook.com/ponys.unina/videos/922838624896130/
[1] D. Brida, C. Manzoni, and G. Cerullo, “Phase-locked pulses for two-dimensional spectroscopy by a birefringent delay line,” Opt. Lett. 37, 3027-3029 (2012) https://doi.org/10.1364/OL.37.003027
[2] S.P. Davis, M.C. Abrams, and J.W. Brault, Fourier Transform Spectrometry (Academic Press, 2001)
[3] A. Perri, B. E. Nogueira de Faria, D. C. Teles Ferreira, D. Comelli, G. Valentini, F. Preda, D. Polli, A. M. de Paula, G. Cerullo, and C. Manzoni, “Hyperspectral imaging with a TWINS birefringent interferometer,” Opt. Express 27, 15956-15967 (2019) https://doi.org/10.1364/OE.27.015956
[4] C. A. Manzoni , D. Brida, G. N. F. Cerullo , US Patent: 9182284 (2015).
[5] A. Perri, B. E. Nogueira de Faria, D. C. Teles-Ferreira, D. Comelli, G. Valentini, F. Preda, D. Polli, A. M. de Paula, G. Cerullo and C. Manzoni, “A hyperspectral camera for conservation science, based on a birefringent ultrastable common path interferometer”. In: Optics for Arts, Architecture, and Archaeology VII. International Society for Optics and Photonics, 2019. p. 110580B. https://doi.org/10.1117/12.2525580
[6] C. Pelosi, A. Lo Monaco, M. Bernabei, G. Agresti, C. Colantonio, A. Perri, D. Comelli, G. Valentini, C. Manzoni, “Beyond the visible: The Viterbo Crucifixion panel painting attributed to Michelangelo Buonarroti,” Microchemical Journal 154, 104636 (2020) https://doi.org/10.1016/j.microc.2020.104636.
[7] A. Candeo, B. E. Nogueira de Faria, M. Erreni, G. Valentini, A. Bassi, A. M. de Paula, G. Cerullo, C. Manzoni, “A Hyperspectral Microscope based on an Ultrastable Common-Path InterferometerA Hyperspectral Microscope based on an Ultrastable Common-Path Interferometer”, APL Photonics, 4, 120802 (2019) doi: https://doi.org/10.1063/1.5129860
Contacts
Prof. Cristian Manzoni, cristian.manzoni@polimi.it; cristianangelo.manzoni@ifn.cnr.it
Prof. Giulio Cerullo, giulio.cerullo@polimi.it